Understanding the Brain’s Response to Sugar Could Help Treat Obesity
Finding new biomarkers to better prevent, diagnose and treat diseases is one of many translational science challenges that NCATS is working to overcome. Biomarkers, such as cholesterol levels, are biological indicators of the presence, absence or stage of a disease. These can help scientists and clinicians better understand diseases, including obesity, and measure a patient’s response to an intervention or treatment.
Although obesity is a growing public health problem, scientists still do not know much about how it develops. Seventy percent of American adults are overweight or obese, and the prevalence of obesity in children is three times higher than just a generation ago. Being overweight increases the risk of many other diseases, including heart disease, Type 2 diabetes, high blood pressure and cholesterol, and stroke. To develop better preventions and treatments, scientists first must understand obesity’s causes. Finding biomarkers for the condition could accelerate this process.
Now, a team of researchers from Yale University, supported in part by a Clinical and Translational Sciences Award (CTSA) from NCATS, have used imaging technology to look at how the brain responds to sugar. This approach could improve scientists’ knowledge of the brain’s role in obesity and could lead to the development of new biomarkers for the condition. Published in the Jan. 2, 2013, issue of JAMA, the imaging study was a multidisciplinary effort involving experts from endocrinology, psychiatry, basic biology, imaging physics, nursing and biostatistics. Team-based approaches to complex translational science problems are at the heart of NCATS science, including in the CTSA program.
Not All Sugar Is the Same
In this case, investigators used brain imaging to reproduce in people what had been observed in mice: different brain responses depending on the type of sugar given. “Work from animal studies suggests a major component of the obesity problem resides in the brain,” explained Robert S. Sherwin, M.D., director of both the CTSA-supported Yale Center for Clinical Investigation (YCCI) and the Yale Diabetes Research Center (YDRC), which is funded in part by NIH’s National Institute of Diabetes and Digestive and Kidney Diseases (NIDDK). “While basic research in animals can provide clues to these differences, this new imaging technique is one of the only ways right now for us to examine the human brain, which is far more complex.”
Using functional MRI (fMRI) to measure blood flow to the brain, researchers can determine which brain areas are active in response to experimental interventions. The team tested 20 adult volunteers to see how their brains responded to both fructose and glucose. Fructose is widely used as a sweetener in foods, and high-fructose diets may contribute to obesity. Compared to glucose, fructose is sweeter and less effective at prompting the body to release the hormone insulin. Insulin acts in the brain to suppress hunger and lessen food’s pleasurable effect. In this way, fructose may increase appetite and eating. In fact, mice given fructose eat more, while glucose has the opposite effect.
To see if humans respond similarly to mice, the researchers scanned the volunteers’ brains twice: once after they drank a glucose drink and once after a fructose drink, both of which contained the same number of calories. They discovered that glucose, but not fructose, reduced brain activity in areas that control appetite and the response to pleasurable aspects of food. The volunteers also reported feeling fuller and more satisfied after drinking glucose. These findings suggest that glucose triggers the brain to reduce the urge to eat.
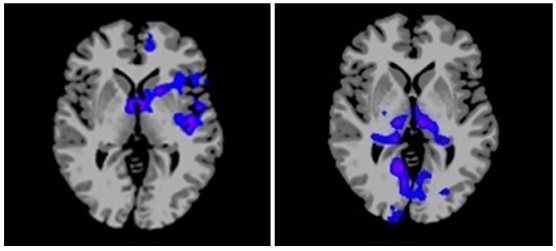
Brain responses to glucose and fructose ingestion show a distinctly different pattern. Glucose reduced blood flow and activity in brain regions that control appetite and reward (shown in blue at left). In contrast, appetite and reward regions remained active after fructose ingestion but activity in memory and sensory perception (shown in blue at right) was suppressed. These images represent composite data from 20 healthy adult volunteers. (Yale University Photo)
Innovation Requires Specialized Support
“The results were really exciting and quite striking,” said Kathleen A. Page, M.D., first author of the study, then a post-doctoral researcher at Yale who now is an assistant professor at the University of Southern California’s Keck School of Medicine. “We were able to translate those animal findings into studies in humans. It’s a perfect example of how translational science should work,” she added.
But it couldn’t have come together without the diverse team of experts and CTSA-supported resources. “So many different elements of the study required special expertise,” Sherwin emphasized. “There is no way a single investigator could do that by himself. These projects require team research from multiple disciplines,” he added.
CTSA-supported services enabled the imaging study at every point along the way. YCCI’s regulatory knowledge specialists helped Page and Sherwin write the study’s protocol so that it met the Institutional Review Board’s (IRB) standards for protection of human subjects. “Their expertise alone significantly reduced the time it took to get the study through the IRB review process,” said Sherwin.
For example, support from the National Cancer Institute (NCI), NIDDK and NCATS’ CTSA program jointly fund Yale’s biostatistical support resource in the Yale Center for Analytical Sciences (YCAS), nursing staff in the Hospital Research Unit (HRU), and technicians in the Core Laboratory. A YCAS statistician performed the study’s data analysis. The YCCI helped recruit volunteers for the study. An HRU dietician prepared the drinks used in the study, while HRU nurses drew blood from the volunteers as they were being imaged. Core Laboratory technicians tested the blood to determine how levels of hormones such as insulin were affected by the sugary drinks. The CTSA also helped fund a critical equipment upgrade to the fMRI machine used in the study.
In addition to support from the YCCI, the research team received support from both NCI and NIDDK. At Yale, the university maximizes its NIH and institutional support for clinical and translational research through joint support of core resources.
Opening Doors for New Knowledge
While the brain study’s findings are consistent with animal studies and other evidence, Sherwin cautions that the imaging technology is still an exploratory method at this stage. “Using fMRI to measure activity gives us a guide for where to look,” Sherwin explained. “Then, we can begin to focus in on these areas and determine what these changes in activity really mean.”
The knowledge gained from this study will clear the path for researchers to delve into exactly how the sugars and feeding-related hormones interact with the brain regions identified in the study. Eventually, this information will clarify how various foods affect over-eating and weight gain, shedding light on the causes of obesity. “When we understand the mechanisms of how the brain responds to sugars, we can begin the process of identifying accurate drug targets and designing drugs and therapies to prevent and treat obesity,” Sherwin said.
Sherwin’s team continues to explore the brain’s role in food and eating. They will soon publish findings of a similar study in which lean and obese adolescents’ brains were scanned while they consumed fructose and glucose. A related study in the same subjects looked at how the brain reacts when a participant views pictures of low and high calorie foods. In both studies, the brains of obese and lean children responded very differently.
“So many chronic health problems begin with obesity. But you have to start somewhere,” Sherwin added. “The new imaging methods enable us to begin that process for the people already suffering from obesity. The knowledge gained also may help policymakers improve dietary recommendations and help public health departments develop better strategies to prevent obesity in healthy children and adults.”
Posted December 2013